Patent-Processor Mac OS
Here is the link to the patent. Thanks to u/freddyt55555 for the heads up on this one. I am extremely excited for this tech. Here are some highlights of the patent: Processor includes one or more reprogrammable execution units which can be programmed to execute different types of customized instructions. Moving forward, I believe Apple will really start taking laptop shares. Their focus will be reaching as many customers as possibles, since they are shifting away from hardware to more services. Hence, iOS apps are able to run on Mac OS, and they are heavily pushing Apple TV+, Apple News, Apple Health and Apple Music.
1. Field of the Invention
The invention generally relates to assigning tasks for processing in a distributed system, and, in particular, to assigning tasks based on a ranking associated with available resources.
2. Description of the Related Art
Distributed computing has become increasingly popular with the maturation of network technology. Oftentimes, it is desirable to exploit the processing power of various networked machines that may otherwise be idle or under utilized. For instace, it may be desirable to use the processing power of the networked machines to compute computationally taxing tasks, such as image processing or rendering, audio processing, video processing, encrypting, decrypting, or the like. One example of a distributed computing architecture is Xgrid™ (Version 1.0) provided by Apple Computer, Inc.
In a typical disturbed computing environment, a central machine on a network divides a project into a number of tasks, which are assigned to one or more of the networked machines for processing or manipulation. The results are then returned to the central machine once the processing is complete.
There are several conventional ways of assigning tasks to volunteer machines. First, tasks may be delegated to pre-determined volunteer machines using a circular, round-robin scheme. In this round-robin approach, incoming tasks are assigned to volunteer machines on a rotating basis in the order those machines are in a list. Second, tasks may be delegated to volunteer machines based on limited information received from these machines regarding their operational capabilities (e.g., processor speed).
Both of these ways can be costly in terms of overhead, and can often produce inefficient results. A round-robin scheme is not particularly efficient for delegating tasks because of the potential mismatch between the amount of work load that is assigned to a particular volunteer machine and its processing capabilities. For example, based on a round-robin scheme, a client machine may delegate a task to a slower, less capable volunteer machine instead of another faster volunteer machine, simply because the slower machine is next in line to receive the task. Similarly, the tasks may be routinely delegated to a volunteer machine that is presently overloaded over an under-utilized volunteer machine based simply on the relative positions of the two volunteer machines in the round-robin scheme.
Like the round-robin scheme, the other scheme (where the controller selects a volunteer machine based on that's machine particular resource capability) also tends to be inefficient and inflexible. This is because the same, fixed criteria (such as speed of the processor) is used to assign tasks to volunteer machines, regardless of nature of the tasks that need to be assigned. For example, a graphics-intensive task that can be more readily processed by a particular graphics card may be assigned to a machine with a faster processor but not the desired graphics card. Similarly, other tasks to be assigned that may not necessarily be suited for volunteer machines that have been identified based on fixed criteria.
Thus, there is a need to efficiently delegate tasks in distributed compilation systems. The present invention is directed to overcoming, or at least reducing, the effects of, one or more of the deficiencies set forth above.
In one aspect of the instant invention, a method is provided for selecting a remote system suitable to process one or more tasks. The method includes transmitting a utility to a plurality of remote systems; receiving ranking values generated by the execution of the utility by each of the plurality of remote systems; and selecting a remote system from the plurality of remote systems to process the task based on the received ranking values.
In another aspect of the instant invention, an apparatus is provided for selecting a remote system suitable to process one or more tasks. The apparatus includes an interface and a control unit. The control unit is adapted to transmit a utility to a plurality of remote systems; receive ranking values generated by the execution of the utility by the plurality of remote systems; and determine one or more remote systems suitable to process a task based on the received ranking values.
In yet another aspect of the instant invention, an article comprising one or more machine-readable storage media containing instructions is provided for selecting a remote system suitable to process one or more tasks. The instructions, when executed, enable a processor to transmit a utility to a plurality of remote systems; receive ranking values generated by the execution of the utility by the plurality of remote systems; and determine one or more remote systems suitable to process a task based on the received ranking values.
In yet another aspect of the instant invention, a distributed compilation system is provided for selecting a remote system suitable to process one or more tasks. The system includes a plurality of remote systems and a controller system. The controller system is adapted to transmit a utility to the plurality of remote systems; receive ranking values generated by the execution of the utility by the plurality of remote systems; and determine one or more remote systems suitable to process a task based on the received ranking values.
The invention may be understood by reference to the following description taken in conjunction with the accompanying drawings, in which like reference numerals identify like elements, and in which:
FIG. 1 is a block diagram of a distributed compilation system, in accordance with one embodiment of the present invention;
FIG. 2 is a block diagram of a client system, a controller system, and/or remote system that may be employed in the distributed system of FIG. 1, in accordance with one embodiment of the present invention; and
FIG. 3 is an illustration of a flow diagram of a rating module executing on the controller system of FIG. 2, in accordance with one embodiment of the present invention; and
FIG. 4 is an illustration of a flow diagram of a delegating module executing on the controller system of FIG. 2, in accordance with one embodiment of the present invention.
While the invention is susceptible to various modifications and alternative forms, specific embodiments thereof have been shown by way of example in the drawings and are herein described in detail. It should be understood, however, that the description herein of specific embodiments is not intended to limit the invention to the particular forms disclosed, but on the contrary, the intention is to cover all modifications, equivalents, and alternatives falling within the spirit and scope of the invention as defined by the appended claims.
Illustrative embodiments of the invention are described below. In the interest of clarity, not all features of an actual implementation are described in this specification. It will of course be appreciated that in the development of any such actual embodiment, numerous implementation-specific decisions must be made to achieve the developers' specific goals, such as compliance with system-related and business-related constraints, which will vary from one implementation to another. Moreover, it will be appreciated that such a development effort might be complex and time-consuming, but would nevertheless be a routine undertaking for those of ordinary skill in the art having the benefit of this disclosure.
Referring to FIG. 1, a distributed system 3 includes a plurality of systems, such as a client system 5, a controller system 7, and remote systems 20, in which tasks may be assigned to one or more of the remote systems 20 by the client system 5 via the controller system 7. The types of tasks that are assigned to the remote systems 20 by the client system 5 may vary, depending on the implementation, and may include, but not be limited to, image processing or rendering tasks, audio processing tasks, video processing tasks, encrypting tasks, decrypting tasks, compilation tasks, or other computationally intensive tasks.
In the illustrated embodiment, the client system 5 provides a task requiring processing to the controller system 7, which may then split the task into one or more sub-tasks and submit them to one or more of the remote systems 20. The remote systems 20, upon executing the tasks or sub-tasks, provide the results to the controller system 7, which then provides the results to the client system 5. Although one client system 5 is illustrated in the distributive system 3 of FIG. 1, it should be appreciated that the distributive system 3 may include a plurality of client systems 5 that submit request tasks to the controller system 7 for processing.
As utilized herein, the term “client” refers to an application (or routine) executing on a system that delegates one or more tasks to other systems for completion. For ease of illustration, the system 5 is designated as the “client” in FIG. 1, although it should be appreciated that any of the remote systems 20 may also be configured as a “client” so that it is able to delegate tasks to the other remote systems 20. Thus, the roles of client and remote systems 5, 20 may vary over time in that the various systems may occasionally take on the role of client and at other times operate as a remote system. It may also be possible that, in some instances, a given system 5, 20 performs a dual role of a client system and a remote system by assigning tasks to other systems 5, 20 and, at substantially the same time, performing tasks for the other systems 5, 20.
It should be appreciated that the three-system configuration (which includes the client, controller, and remote systems 5, 7, and 20) shown in FIG. 1 is exemplary, and that in alternative embodiments, other configurations may be used without deviating from the spirit and scope of the present invention. For example, in an alternative embodiment, the functionality of these systems 5, 7, and 20 can be combined or merged with one another. For instance, in one embodiment, the client system 5 may perform the role of the client system 5 as well as the controller system 5. As such, this configuration would include a client system 5 that communicates with the remote systems 20 without a separate, intermediary controller system 7.
The client system 5, the controller system 7, and remote systems 20, in one embodiment, can be coupled to each other by a data network (not shown), which may be a public or a private network. Examples of the data network may include local area networks (LANs), wide area networks (WANs), intranets, the Internet, or the like. The data network may be a packet-switched data network, such as a data network according to the Internet Protocol (IP). A “data network” may refer to one or more channels, links, or paths, and systems or devices (such as routers) used to route data over such networks, channels, links, or paths. If desired, client system 5 and controller system 5 may, in one embodiment, may multicast data packets to the remote systems 20.
The systems 5, 7 and 20 may be any processor-based systems, such as computers in the form of desktops, laptops, mainframes, personal digital assistants, or the like. In one embodiment, the systems 5, 7, 20 may be located at various locations 23, which may be representative of different departments or centers of an organization, or, alternatively, different offices of an organization. Thus, for example, the locations 23, in one embodiment, may represent different offices/centers within a building, within one or more building complexes, within a city or country, or the like.
As described below, in accordance with one embodiment of the present invention, the controller system 7 associates ranking information with the plurality of remote systems 20, and this ranking information is then utilized to identify remote systems 20 that are suitable to process task(s) provided by the client system 5. In general, remote systems 20 are “ranked” based on a ranking utility associated with a task. The ranking utility, which may be an executable routine or a runnable script, includes a criteria (or algorithm) that determines if the remote system 20 is adequately equipped with resource(s) to perform the task provided by the client system 5. The criteria may be based on definitive criteria (such as hardware configuration of a remote system 20), more fluid criteria (such as the operational load of the remote system 20 at a given time), or a combination of both. The assigner of the task selects the criteria that are pertinent to the task at issue such that the remote systems 20 that match closest to the criteria will have a higher rank relative to those that do not. In one embodiment, the ranking values can be scaled (e.g., scaled to a range between 0 to 100, with 100 being the highest ranking, or vice-versa).
Patent-processor Mac Os 11
As noted, the generated ranking values of the various remote systems 20 can then be utilized to determine which of the remote systems 20 are suitable to assist with processing the submitted task provided by the client system 5. In one embodiment, aside from generating a ranking value, the ranking utility may also provide additional information (referred to as “metadata” herein) about the ranking value or the remote system 20. For example, in addition to the ranking value, the ranking utility may indicate variety of information about the remote system 20, such as the amount of configured memory (e.g., 12 gigabytes), which version of the relevant software is installed, the level of processor speed (e.g., 3 gigahertz), or the like. In other embodiments, the metadata can indicate if the resources of the remote system 20 exceed at threshold value, such as whether the configured memory exceeds a certain threshold, whether the amount of available hard disk space is at least a certain specified value, whether the processor speed is about a selected value, or the like. This metadata, in one embodiment, can be used to further refine which remote systems 20 are better suited than other qualified systems to perform the task to be assigned.
One or more embodiments of the present invention allow an assignor of a task (such as the client system 5, in this case) to efficiently and effectively identify and assign tasks to one or more remote stations 20. This is because the task assigner has the option to define its own criteria to identify remote systems 20 that are better equipped to process the task at hand. Moreover, because the defined criteria can be embodied in a ranking utility that can be executed by the remote machines 20, the task assignor need not know in advance the configuration of the remote systems 20; rather, this information can be obtained when the ranking utility is executed by the remote systems 20. Additionally, the use of the ranking utility also makes it possible to collect up-to-date configuration information (or the current conditions) of the remote machines 20.
In the illustrated embodiment, the client system 5 includes an application module 24 that provides one or more tasks to the controller system 7 to delegate to the qualified remote systems 20. In one embodiment, the application module 24 also provides at least one ranking utility 25 that each remote system 20 can execute to generate its ranking value. The ranking value can be used to determine whether a given remote system 20 is suitable to participate in the execution of tasks. In one embodiment, the client system 5 may include more than one ranking utility, each embodying an algorithm or criteria useful in identifying remote systems 20 that are suitable to perform tasks assigned by the client system 5.
In the illustrated embodiment, the client system 5 transmits the ranking utility 25 to the controller system 7, which in turn manages the distribution of the utility 25 to the remote systems 20. In an alternative embodiment, the client system 5 may transmit its ranking utility 25 to one or more of the remote systems 20 without an intervening controller system 7. The manner in which the ranking utility 25 is provided to the remote systems 20 is implementation specific, and thus can vary based on the designer's desires or goals. In some instances, the ranking utility 25 may be preinstalled or manually installed on the remote systems 20 and thus it may not be necessary to transmit a copy of the ranking utility 25.
As noted, the application module 24 of the client system 5 provides one or more tasks that require completion. In one embodiment, in connection with submitting task(s), the application module 24 of the client system 5 also provides an identifier to the controller system 7. The identifier specifies the particular requirements of processing the task. For example, the identifier may indicate the ranking utility that is associated with the incoming task so that the appropriate ranking values can be utilized to determine which remote stations 20 are suitable to participate in the execution of the submitted task.
In the illustrated embodiment, the controller system 7 includes a rating module 26 that determines the ranking of the various remote systems 20 based on the ranking utility 24 provided by the client system 5. The controller system 7 also includes a delegating module 27 that assigns tasks (or sub-tasks) to the remote systems 20 based on the determined ranking values of the remote systems 20.
In the illustrated embodiment of FIG. 1, the remote systems 20 include a daemon module 35, which executes on the remote systems 20, and responds to requests from the client system 5. For example, the daemon module 35 accepts the ranking utility 25 from the controller system 7, executes that ranking utility 25, and provides the results (e.g., ranking value) to the controller system 7. Although not shown, in one embodiment, the client system 5 may also include the daemon module 35.
In the illustrated embodiment, the daemon module 35 utilizes a processing module 40 executing on the remote system 20 to complete the tasks that are assigned to the remote system 20. In the context of a graphics-based task, the processing module 40 performs the appropriate calculations and provides the results to the controller system 20, which in turn can provide the results to the application module 24 of the client system 5. As an additional example, in the context of a code compilation task, the processing module 40 may, for example, compile one or more source files to produce object code files, link files with object code segments to produce executable files, perform pre-processing tasks, assemble files, or the like, and then provide the results for the client system 5.
The application module 24, rating module 26, delegating module 27, daemon module 35, and processing module 40, in the illustrated embodiment, are implemented in software. While these modules 24, 26, 27, 35, and 40 are illustrated as four distinct modules for the purposes of this discussion, it should be appreciated that some or all portions of these modules may be combined or expanded into any number of module(s). The modules 24, 26, 27, 35, and 40 in the illustrated embodiment are executable on the systems 5, 7, and 20, each of which may be, for example, a laptop computer, a desktop computer, a mainframe computer, a handheld device, or any other processor-based system capable of executing instructions. In alternative embodiments, some or all portions of one or more of these modules 24, 26, 27, 35, 40 may be implemented in hardware or firmware.
Referring now to FIG. 2, a stylized block diagram of a system 200 is illustrated, in accordance with one embodiment of the present invention. The system 200 may be implemented as the client system 5, controller system 7, and/or remote systems 20 of FIG. 1. The system 200 comprises a control unit 215, which in one embodiment may be a processor, and is capable of interfacing with a north bridge 220. The north bridge 220 provides memory management functions for a memory 225, as well as serves as a bridge to a peripheral component interconnect (PCI) bus 230. In the illustrated embodiment, the system 200 includes a south bridge 235 coupled to the PCI bus 230.
A storage unit 250 is coupled to the south bridge 235. A variety of modules, such as the application module 24, rating module 26, delegating module 27, daemon module 35, and processing module 40, may be stored in the storage unit 250 and executed by the control unit 215. Additionally, the ranking utility 25 may also be stored in the storage unit 250. Although not shown, it should be appreciated that in one embodiment an operating system, such as Windows®, Disk Operating System®, Unix®, Linux®, MAC OS®, or the like, may be stored on the storage unit 250 and executable by the control unit 215. The storage unit 250 may also include device drivers for the various hardware components of the system 200.
In the illustrated embodiment, the system 200 includes a display interface 247 that is coupled to the south bridge 235. The system 200 may display information on a display device 248 via the display interface 247. The south bridge 235 of the system 200 may include a controller (not shown) to allow a user to input information using an input device (not shown), such as a keyboard and/or a mouse.
The south bridge 235 of the system 200, in the illustrated embodiment, is coupled to a network interface 260, which may be adapted to receive, for example, a local area network card. In an alternative embodiment, the network interface 260 may be a Universal Serial Bus interface or an interface for wireless communications. The system 200 communicates with the remote system 20 coupled to a data network through the network interface 260.
It should be appreciated that the configuration of the system 200 of FIG. 2 is exemplary in nature and that, in other embodiments the system 200 may include fewer, additional, or different components without deviating from the spirit and scope of the present invention. For example, in an alternative embodiment, the system 200 may not include a north bridge 220 or a south bridge 235, or may include only one of the two bridges 220, 235, or may combine the functionality of the two bridges. As another example, in one embodiment, the system 200 may include more than one control unit 215. Similarly, other configurations may be employed consistent with the spirit and scope of the present invention.
Referring now to FIG. 3, a flow diagram of one or more acts that are performed by the rating module 26 of the controller system 7 is illustrated, in accordance with one embodiment of the present invention. In particular, FIG. 3 illustrates one embodiment of a method for identifying the remote stations 20 that are suitable to perform the task(s) submitted by the client system 5. As noted earlier, the ranking values are calculated when the remote stations 20 execute the ranking utility provided by the client system 5. It should be appreciated that, in one embodiment, the client system 5 may provide the ranking utility 25 to the controller system 7 contemporaneously with the task it needs completed, or, alternatively, provide it separately from the task. For ease of illustration, it is assumed that in FIG. 3, the client system 5 provides the ranking utility 25 in advance of the task.
In FIG. 3, the rating module 26 of the controller system 7 receives (at 310) at least one ranking utility 25 (or a copy of the ranking utility 25) from the application module 24 of the client system 5. It should be appreciated that, in one embodiment, a plurality of client systems 5 may each transmit its own ranking utility (or utilities) to the controller system 7. Thus, at any given time, the controller system 7 may be handling a plurality of ranking utilities from a plurality of sources. However, for ease of illustration, it is assumed that one client system 5 transmits the ranking utility 25 (or utilities) to the controller system 7. It should be appreciated that, in one embodiment, the client system 5 may transmit a plurality of different ranking utilities 25 to the controller system 7.
The rating module 26 of the controller system 7 stores (at 310) the received ranking utility 25. The act of storing the received ranking utilities may include storing (at 312) an authenticating value associated with each of the ranking utilities. This authenticating value may be utilized to determine if the previously-stored ranking values are still valid. For example, if the authenticating value of a newly received ranking utility matches that of a previously received ranking utility, then that is an indication that the ranking values collected based on the previously received ranking utility are still valid. As such, the rating module 26 of the controller system 7 need not collect any new ranking values and need not overwrite the previously-received ranking utility. The authenticating value, in one embodiment, may be a hash value or a checksum value for allowing comparison of newly received ranking utility to a previously stored ranking utility.
As part of storing (at 310) the received ranking utility, the rating module 26 of the controller system 7 may also store (at 316) a timestamp of the last time a client system 5 submitted a task to the controller system 7 so that any previously-submitted, older ranking utilities can be removed after some period of idleness. In one embodiment, the rating module 26 updates the timestamp associated with the ranking utility each time the ranking values associated with the ranking utility are used to identify suitable remote stations 20 for processing a received task.
The rating module 26 of the controller system 7 provides (at 320) the received ranking utility 25 (or a copy of the ranking utility 25) to the one or more available remote systems 20 for execution. In an alternative embodiment, the rating module 26, if the controller system 7 receives a plurality of different ranking utilities from the client system 5, may be provide these ranking utilities to the remote systems 20. In one embodiment, the controller system 7 provides the ranking utility 25 to the remote systems 20 as the systems become available or otherwise establish a communication link with (or bind to) the controller system 7. In an alternative embodiment, the rating module 26 of the controller system 7 may multicast a notification to the remote systems 20, which may be communicatively linked to the controller system 7 via a data network, that a ranking utility is available. In a multicasting embodiment, the controller system 7 announces to a router (not shown) that a ranking utility is available for transmission. The router in turn multicasts the announcement to the available nodes or remote systems 20 based on the remote systems 20 identified in a multicast group or distribution list. The remote systems 20, in response to receiving the notification, can retrieve the ranking utility 25. In one embodiment, the router may dynamically update the contents of its multicast group. That is, as remote systems 20 become available or inaccessible, the router updates its multicast group accordingly. In one embodiment, the multicast group or distribution list may contain destination addresses associated with each of the remote systems 20 included in the group or list. The router, in one embodiment, may substantially simultaneously indicate to the available remote systems 20 regarding the availability of task(s). In one embodiment, the router may multicast the task notification to each of the available remote systems 20 using an efficient routing path.
Upon reception of the ranking utility, each of the remote systems 20 can execute its ranking utility 25 and provide the resulting ranking value to the controller system 7. The rating module 26 of the controller system 7 receives (at 330) results from the remote systems 20 that execute the ranking utility 25. The results returned will depend on the criteria specified in the ranking utility 25. In one embodiment, the results received will include the ranking value (see block 332) from the remote systems 20. In an alternative embodiment, the results received may also include metadata (see block 334) about the ranking value or the remote systems 20. The rating module 26 of the controller system 7 stores (at 340) the results that are received. These stored results can be utilized to delegate tasks to the remote systems 20, as described below.
FIG. 4 illustrates a flow diagram of the delegating module 27 of the controller system 7 for assigning task(s) to the remote system 20, in accordance with one embodiment of the present invention. For ease of illustration, it is assumed that the controller system 7 has previously obtained the ranking values from the various remote systems 20 in the distributed system 3 of FIG. 1. One manner of obtaining the raking values is described above in connection with FIG. 3.
In FIG. 4, the delegating module 27 receives (410) information regarding at least one task requiring processing from the application module 24 of the client system 5. In one embodiment, the received information may include information about the task itself (see block 412). In one embodiment, the received information may also include an identifier (see block 414) that specifies the requirements for processing the task. For example, the identifier may indicate that the ranking values generated using a particular ranking utility should be used when determining which of the remote systems 20 are qualified to process the received task. In one embodiment, the identifier may indicate that ranking values from two or more ranking utilities should be combined in determining remote systems 20 that are suitable to process the received task.
The delegating module 27 determines (at 420) if the ranking values that are to be used are current or valid. The ranking values may not be valid for one of a variety reasons. For example, the lifetime of the ranking values may have expired such that they may not reflect current conditions of the remote systems 20. This may be particularly true for ranking values that are based on transient characteristics such as a remote system's current load or the quality of a network connection to that remote system. Another reason the ranking values may not be valid is if the ranking utility 125 that was executed to generate these values is outdated (either because a newer ranking utility has been received or because the lifetime of that ranking utility has expired). Similarly, there may be other reasons the ranking values may no longer be current or valid. In FIG. 4, if it is determined (at 420) that the ranking values are not current, the delegating module 27 updates (at 425) the ranking values. These values may be updated, for example, by requiring the remote stations 20 to execute the ranking utility 125 and provide the updated ranking values.
If it is determined (at 420) that the ranking values stored on the controller system 7 are current or valid, or if the invalid ranking values have been updated (at 425), the delegating module 26 identifies (at 430) which remote systems 20 are suitable or qualified to process the received task based on the results received from the execution of the ranking utility by the remote systems 20 (see blocks 330, 332, and 334 of FIG. 3). As shown in block 330-334 of FIG. 3, the results may include the ranking value, as well as metadata associated with that ranking value. Thus, in one embodiment, the delegating module 26 may determine that that only those remote stations 20 having a ranking value above a selected threshold level are qualified to process the task.
In another embodiment, the ranking value and the associated metadata may both be utilized to identify which of the remote systems 20 qualify to process the received task. For example, the delegating module 26 may initially use the ranking value to identify a select number of remote systems 20 that are qualified to process the received task. From this initial group of remote systems 20, the delegating module 26 may further narrow the number of qualifying remote systems 20 based on the received metadata. For instance, assuming that the metadata returned by each of the remote systems 20 related to the amount of available memory (e.g., 12 gigabytes) in that remote system 20, then only those remote systems 20 that have requisite amount of available memory would be qualified to execute the task. It should be appreciated that the ‘memory’ metadata example provided herein is illustrative only, and that, in alternative embodiments, any variety type of metadata may be employed to allow the task assignor greater flexibility in identifying suitable remote systems 20 to process the task.
Once the remote systems 20 that are suitable to perform the task have been identified (at 430), the delegating module 26 assigns (at 440) the task to at least one of the identified remote system 20. If the entire task is to be assigned to a single remote system 20, the delegating module 26 may select, for example, the remote system 20 with the highest ranking value among the qualifying remote systems 20. If the task is to be broken into several sub-tasks, the delegating module 26 may select, for example, from among those qualifying remote systems 20 that have the highest ranking values.
Once the task or sub-tasks are assigned, the responsible remote stations 20 execute the assigned task (or sub-task) and return the results to the delegating module 26 of the controller system 7. The delegating module 26, upon receiving (at 450), provides (at 460) the results to the application module 24 of the client system 5.
The foregoing description describes one or more embodiments for efficiently and effectively identifying one or more remote systems 20 in a distributed system 3 that are better suited to perform task(s) needing completion. In one illustrated embodiment, the task submitter is allowed to specify criteria in the form of a ranking utility that, when executed by each remote system 20, returns a ranking value for that remote system 20. The ranking value provides a basis to determine which of the remote systems 20 are adequately equipped to handle the task being assigned. Thus, one or more embodiments of the present invention allow the task submitter to specify prerequisite conditions for performing a task and allow the remote systems 20 to indicate by way of a ranking utility as to whether the systems meet those conditions. The use of the ranking utility also provides the task submitter a dynamic way to determine current (or up-to-date) operating conditions (e.g., available memory, network latency to a particular server or disk, etc.) of the remote systems 20 that are available to assist with processing the task.
Those skilled in the art will appreciate that the various system layers, routines, or modules illustrated in the various embodiments herein may be executable control units (such as the control unit 215 (see FIG. 2)). The control unit 215 may include a microprocessor, a microcontroller, a digital signal processor, a processor card (including one or more microprocessors or controllers), or other control or computing devices. The storage devices 250 referred to in this discussion may include one or more machine-readable storage media for storing data and instructions. The storage media may include different forms of memory including semiconductor memory devices such as dynamic or static random access memories (DRAMs or SRAMs), erasable and programmable read-only memories (EPROMs), electrically erasable and programmable read-only memories (EEPROMs) and flash memories; magnetic disks such as fixed, floppy, removable disks; other magnetic media including tape; and optical media such as compact disks (CDs) or digital video disks (DVDs). Instructions that make up the various software layers, routines, or modules in the various systems may be stored in respective storage devices. The instructions when executed by a respective control unit 215 causes the corresponding system to perform programmed acts.
The particular embodiments disclosed above are illustrative only, as the invention may be modified and practiced in different but equivalent manners apparent to those skilled in the art having the benefit of the teachings herein. Furthermore, no limitations are intended to the details of construction or design herein shown, other than as described in the claims below. It is therefore evident that the particular embodiments disclosed above may be altered or modified and all such variations are considered within the scope and spirit of the invention. Accordingly, the protection sought herein is as set forth in the claims below.
The present invention relates generally to call processor architectures, and in particular to methods and apparatus for high-availability call processor systems and methods.
Conventional call processor systems use special purpose, dedicated systems for implementing redundancy. For example, some conventional systems utilize two computer systems, one of which is active and the other standby, and special purpose hardware and software that interacts with each computer system to implement high-availability. The special purpose hardware and software communicates with the active computer system to capture status information so that in the event the active system goes down the standby system can start in place of the active system using the information collected by the special purpose hardware and software.
Thus, conventional high-availability architectures require special purpose hardware and software, which raises system costs. The additional costs make systems very expensive. There is, therefore, a need for a high-availability architecture that solves the problems associated with special purpose hardware and software high-availability systems.
The call processor system consistent with the invention takes advantage of commercial off the shelf (COTS) products while maintaining high availability. The call processor system is comprised of four main components: X86 Single Board Compute Platform (CP PII), System Area Network (SAN) interface, high-speed pipes (HSP's), System Utility Card (SU), and a Core to Network Interface Card (cCNI).
The compact peripheral component interconnect (cPCI) standard specifies both the packaging, format and the electrical interface for the back plane and the on card bus structure of the system. The cPCI standard also include the hotswap specification. The hot swap specification has standardized a way to insert and remove circuit packs in a live system without affecting other circuit packs that share the same bus. The specification also specifies how an operating system (OS) can be notified so as to allow dynamic loading of drivers.
By using COTS components, future upgrades to newer technologies are easy and quick, thus leveraging the time to market opportunities that can be gained by using, third party off the shelf components. The system implements a high-availability architecture based around a system area networks (SAN) technology that allows the call processor to perform better than conventional systems, and also build a system that could easily evolve. The system features include a 1+1 sparing strategy, graceful switchover that preserves all call states, ungraceful switchover in case of node failure that would preserve all existing, established calls, hard disk drive redundancy, and IP take over (the system presents a logical Ethernet connection to the OA&M connection). The system addresses all of these issues and in addition provides for querying the health of the inactive side at any time. Due to the high bandwidth of new high-speed network interface cards, the complete system memory image can be transferred to the inactive system on command within one second; this type of performance allows the active processor to run asynchronously to the standby system, allowing significant performance gains to be achieved on the active side. When a graceful switchover is required, the complete system state of the active processor can be transferred to the standby processor allowing the standby processor to continue as if it had been in control all the time. This strategy works well for hardware failure.
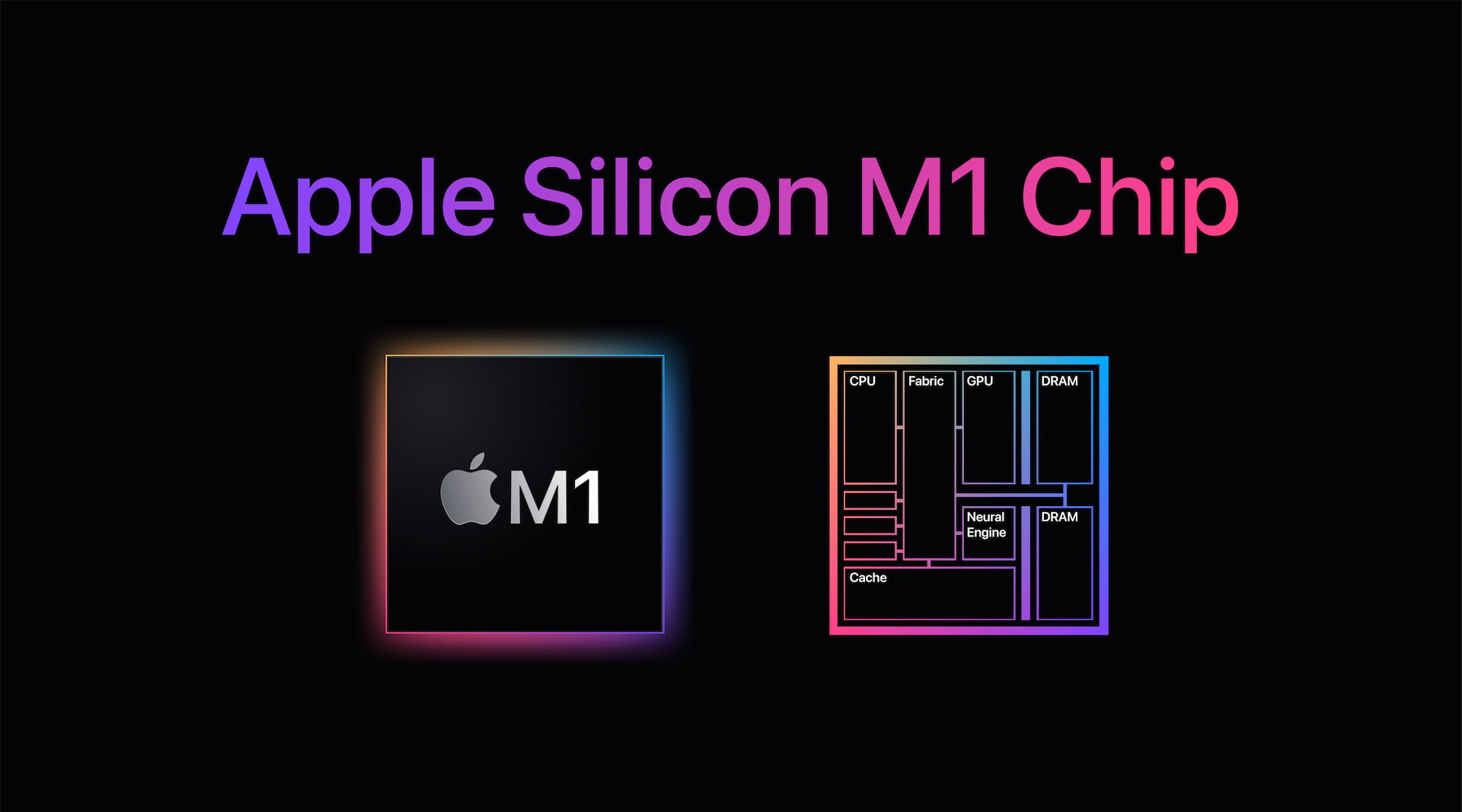
In a redundant system, one side is in active mode running call processing, and the other side is in standby mode ready to take over. This is called a redundancy approach. Both the active side and the standby side are running, in parallel, however, the active side is running call processing applications while the inactive side is running only diagnostics and management tasks. Since the status of the inactive side is always known, the system can make better decisions on whether or not to switchover. Redundancy control in a call processor consistent with the present invention is software-based. As a result, all the redundancy related functions are implemented by software. This has several advantages, such as loosening the coupling between the two sides, thus reducing adverse interference between them. Certain features of the redundancy control may still be implemented in hardware.
First cPCI shelf
Network
PCI bus
ISA bus
Application adaptors layer
Hardware abstraction layer
BSP
Exception and interrupt handling mechanism of the Real-time OS Kernel
Communication stacks
OS extensions
Communication Manager
LCS
From synch on the block R/A, R/I, LCS
From update, the system may move to state HSP down if it is determined that the high speed pipe is down. From HSP down, the system may move back to synch if the high speed pipe comes back up.
The system may also move from update in block R/A, R/I to update in block R/I, R/A if there is a graceful switchover active (g-swoA). For example, there might be a graceful switchover to the other system for routine maintenance. The system may move back to the update in block R/I, R/A if there is a graceful switchover inactive (g-swoI).
From block R/I, R/A, there may be an ungraceful switchover (ug-swoI), which moves the system to the synch state in block R/A, R/I.
From switch state, LCS
From synch in block R/I, R/A, if a split inactive (splitI) is performed the system moves to S/A in state S/I, S/A.
Heart beat
In one embodiment, two pipes are used for vote and heartbeat messaging: High Speed Pipe and Ethernet. Two pipes have several advantages, including: (1) eliminating single point of failure to increase system reliability, and (2) avoiding unnecessary ungraceful switchover when the HSP pipe fails.
SWOMgr
An ungraceful switchover occurs when a more catastrophic event occurs, such as when CP PII
LCS
PDataMgr
Disk redundancy
During disk synchronization all updates (writes and iocti calls that modify the disk) on the protected and unprotected partitions will be mirrored to the slave CP when the system is in redundant mode.
Redundancy state is stored in LCS
In split mode, the system behaves a little differently. In this scenario, there are two active nodes, each totally independent. When the nodes are in split mode, they both only use their individual protected and unprotected partitions. The independent partition is not used. The redundancy agent does not propagate update I/O because the system is in split mode.
A multiple partitioning scheme is implemented that provides three separate partitions on the same hard disk. It can set up the disk into standard MS-DOS format partitions. In the CPP model, three partitions are setup according to the following table:
Side | Partition requested | Partition used |
Master | /p | /C |
Master | /u | /D |
Slave | /p | /C |
Slave | /u | /E |
The partitioning scheme also allows an application to retrieve the partition information from the disk. This includes the number of partitions, the sizes of each, where they begin/end, and the type of file system located on each. The users of disk redundancy only include other software modules.
Initialization of disk redundancy
The disk redundancy system consistent with the principles of the present invention has several advantages, including: providing high data availability with low performance overhead, keeping the cost low by providing a design that does not depend on customized hardware, and minimizing modifications to the existing software by providing application-transparent disk redundancy software.
In one embodiment, disk redundancy is built on an IDE controller which comes with the CP card. As a result, no additional cost is required and no change is necessary to the existing hardware.
Data mirroring between the two disks is achieved via messaging over a high speed pipe. This same high speed pipe is used for protected memory mirroring, heartbeat, and switchover. There are several advantages of this approach, including: (1) two CPU nodes can be physically located at much farther distances, and (2) combined with the loosely-coupled CPU redundancy strategy, the redundant system will become a message-based system, which is advantageous for moving toward distributed computing.
Mac Os Processor
In one embodiment, disk redundancy software is built on top of the disk device driver and under the DOS file system. This approach provides simplicity. The software is self-contained, independent of the file system, and there is no need to manage file system states during switchover, split and join operations.
Disk redundancy software in each side of the redundant system reports faults to the fault handling module of its own side. The disk redundancy software itself does not exchange fault information between the two sides. One advantage of this approach is that general fault handling mechanism should be the same for both simplex and duplex systems, and allow the logical call server to handle redundancy specific decisions. This also has the advantage that the logical call server module is the only module that has the information of both sides.
To effectively handle the differences in traffic requirements between disk redundancy, stop and copy, and the heartbeat/checkpointing facilities, in one embodiment three separate channels are implemented across the high speed pipe.
IP management
To facilitate communication with external systems running on a LAN, the present invention is equipped with two Ethernet ports, which are connected to the LAN. Thus, certain IP management functionalities are provided. Since both nodes are running, two IP addresses allow external systems to talk to both nodes even though the system is addressed using a single IP address. An IP address is not assigned to a physical node (node
Gratuitous address resolution protocol (ARP) handles run-time IP address change. When a host's IP address is changed, it broadcasts its IP address and its MAC address to the network. Other nodes on the network can continue to communicate with the host. In the present invention, gratuitous ARP is used during a graceful switchover. Graceful switchover copies the entire memory image from the old active side to the new active side. As a result, the new active side gets the active-IP-address but it still has the existing MAC address. To inform this change the new active side sends a gratuitous ARP as one of its post-switchover actions. The gratuitous approach is useful in a multi-node architecture. During the post-switchover, the new inactive side sets its IP address to the inactive P-address by calling a function, such as VxWork's if AddrSet( ), which does gratuitous ARP.
The new active side goes through a warm restart to set its IP address to active-IP-address. DRAM
In true redundant state
Split mode is used when it is desirable to break redundancy in a controlled way. Split is used when one side needs to be brought down, such as for maintenance. In split state
In single redundant not ready state
Node
Node
For node
Continuing with the step
For node
For node